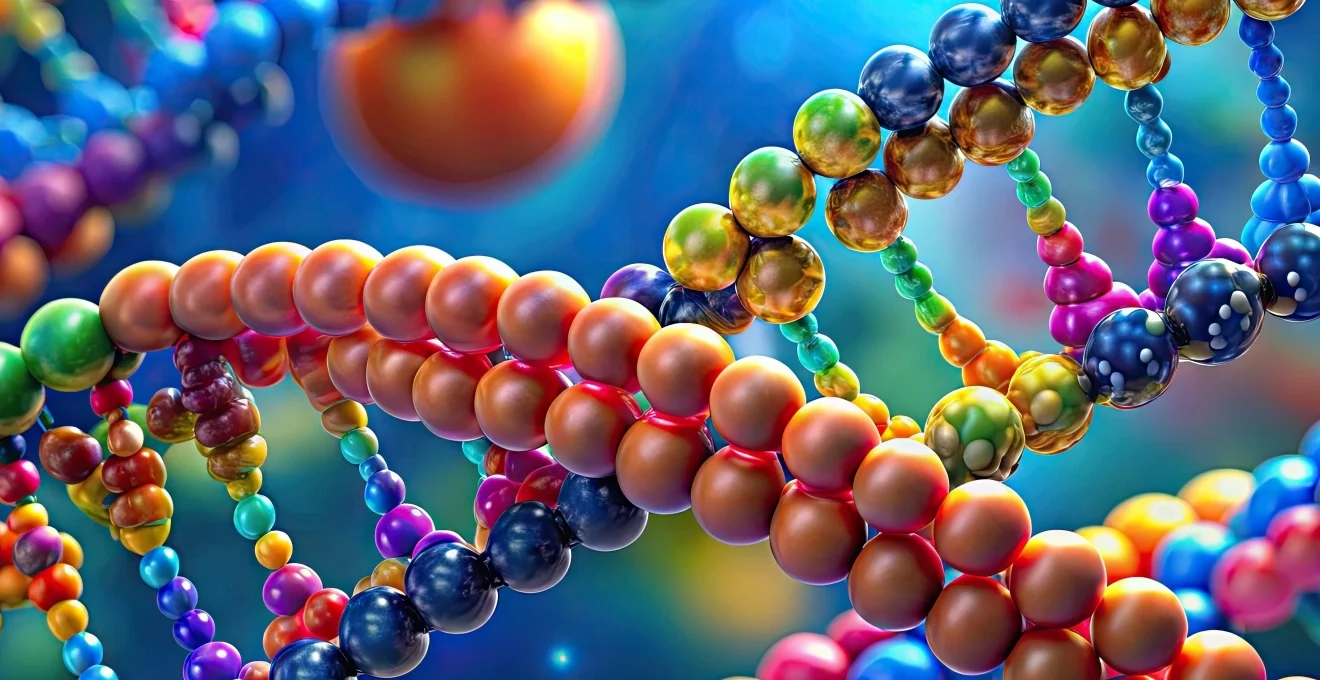
In the rapidly evolving field of molecular diagnostics, aptamer technology has emerged as a powerful tool for detecting and quantifying biomarkers with unprecedented precision. These short, single-stranded nucleic acid molecules have revolutionized our approach to disease detection and monitoring. The ability to label aptamers with various chemical moieties has greatly expanded their utility in diagnostic applications, offering high sensitivity and specificity in complex biological samples.
As we delve into the intricate world of aptamer labeling, we'll explore the fundamental principles that make these molecules so versatile, the cutting-edge techniques used to modify them, and the innovative applications that are shaping the future of medical diagnostics. From fluorescent tags to nanoparticle conjugates, the science behind aptamer labeling is opening new frontiers in personalized medicine and point-of-care testing.
Fundamentals of aptamer technology in diagnostic applications
Aptamers are synthetic oligonucleotides that can bind to specific target molecules with high affinity and selectivity. Their unique three-dimensional structures allow them to recognize a wide range of targets, from small molecules to proteins and even whole cells. This versatility makes aptamers ideal candidates for diagnostic applications, where they can serve as molecular probes for detecting biomarkers associated with various diseases.
The key to aptamer functionality lies in their ability to form complex secondary and tertiary structures through intramolecular interactions. These structures create binding pockets that are complementary to their target molecules, allowing for highly specific recognition. Unlike antibodies, which are produced biologically, aptamers are chemically synthesized, offering several advantages in terms of production, stability, and modification potential.
One of the most significant benefits of aptamers in diagnostics is their ability to be easily modified with various labels without significantly affecting their binding properties. This characteristic is crucial for developing sensitive and specific diagnostic assays. Labeling aptamers allows for the detection and quantification of target molecules using a variety of analytical techniques, ranging from simple colorimetric tests to advanced imaging methods.
Chemical modifications for aptamer labeling
The process of aptamer labeling involves the attachment of specific chemical groups or molecules to the aptamer sequence. These modifications serve multiple purposes, including enhancing detection sensitivity, improving stability, and enabling various detection modalities. The choice of labeling strategy depends on the intended application and the detection method to be employed.
Fluorescent dye conjugation techniques
Fluorescent labeling is one of the most commonly used techniques for aptamer modification. This method involves attaching fluorescent dyes to the aptamer, typically at the 5' or 3' end. The process of conjugating fluorescent dyes to aptamers requires careful consideration of the dye's properties and the aptamer's structure to ensure that the labeling does not interfere with target binding.
Several fluorescent dyes are popular for aptamer labeling, including:
- Fluorescein derivatives (e.g., FAM, FITC)
- Cyanine dyes (e.g., Cy3, Cy5)
- Alexa Fluor dyes
- ATTO dyes
These dyes offer a range of excitation and emission wavelengths, allowing for multiplexed detection of multiple targets simultaneously. The choice of dye depends on factors such as quantum yield, photostability, and compatibility with available detection instruments.
Biotin-streptavidin systems for aptamer detection
The biotin-streptavidin system is another powerful tool for aptamer labeling and detection. This approach takes advantage of the extremely strong and specific interaction between biotin and streptavidin. Aptamers are typically modified with biotin during synthesis, which can then be detected using streptavidin conjugated to various reporter molecules.
The biotin-streptavidin system offers several advantages:
- High sensitivity due to the strong biotin-streptavidin interaction
- Versatility in detection methods (e.g., colorimetric, fluorescent, enzymatic)
- Ability to amplify signals through multiple biotin-streptavidin interactions
- Compatibility with a wide range of analytical platforms
This labeling strategy is particularly useful in applications such as ELISA-like assays, where the biotin-labeled aptamer can be captured on a streptavidin-coated surface and subsequently detected using various reporter systems.
Radioactive isotope labeling methods
While less common due to safety concerns and regulatory requirements, radioactive labeling of aptamers remains a valuable technique for certain research and diagnostic applications. This method involves incorporating radioactive isotopes into the aptamer structure, typically through the use of radiolabeled nucleotides during synthesis or post-synthetic modification.
Common isotopes used for aptamer labeling include:
- 32P for high-energy beta emission
- 35S for lower-energy beta emission
- 125I for gamma emission
Radioactive labeling offers exceptional sensitivity, making it useful for detecting low-abundance targets. However, the short half-life of many isotopes and the need for specialized handling and disposal procedures limit its widespread use in clinical diagnostics.
Nanoparticle-based aptamer functionalization
The integration of aptamers with nanoparticles has opened up exciting new possibilities in diagnostic applications. Nanoparticles, such as gold nanoparticles (AuNPs), quantum dots, and magnetic nanoparticles, can be functionalized with aptamers to create highly sensitive and versatile detection systems.
Aptamer-nanoparticle conjugates offer several advantages:
- Enhanced signal amplification due to the properties of nanoparticles
- Multivalent binding for improved affinity and specificity
- Potential for multimodal detection (e.g., optical and magnetic)
- Compatibility with various detection platforms, including lateral flow assays
The functionalization process typically involves attaching aptamers to the nanoparticle surface through covalent bonding or non-covalent interactions. The resulting conjugates can be used in a wide range of diagnostic applications, from simple colorimetric tests to advanced imaging techniques.
Advanced aptamer selection processes for precision diagnostics
The effectiveness of aptamer-based diagnostics relies heavily on the quality and specificity of the aptamers used. Advanced selection processes have been developed to generate aptamers with optimal binding properties for diagnostic applications. These methods aim to identify aptamers that not only bind their targets with high affinity and specificity but also perform well in complex biological matrices.
SELEX (systematic evolution of ligands by exponential enrichment) methodology
SELEX is the foundational method for aptamer selection and remains a crucial technique in the field. This iterative process involves exposing a large library of random oligonucleotide sequences to the target of interest, followed by washing away non-binding sequences and amplifying the bound sequences. Through multiple rounds of selection and amplification, aptamers with high affinity and specificity for the target are enriched.
Key steps in the SELEX process include:
- Incubation of the oligonucleotide library with the target
- Separation of bound from unbound sequences
- Elution of bound sequences
- Amplification of selected sequences
- Generation of a new, enriched library for the next round
Modern SELEX techniques often incorporate negative selection steps to remove sequences that bind to undesired targets, improving the specificity of the resulting aptamers.
Cell-selex for Whole-Cell target recognition
Cell-SELEX is a variation of the SELEX process that uses whole cells as targets for aptamer selection. This method is particularly valuable for diagnostic applications where the specific molecular target may be unknown or where the goal is to identify aptamers that recognize cell surface markers in their native conformation.
Cell-SELEX offers several advantages for diagnostic aptamer development:
- Selection of aptamers against complex targets in their natural state
- Potential for discovering new biomarkers
- Generation of aptamers suitable for cell-type specific targeting
- Compatibility with live cell imaging and flow cytometry applications
The process typically involves alternating positive selection against the target cell type and negative selection against control cells to ensure specificity. The resulting aptamers can be used for various diagnostic applications, including cancer cell detection and pathogen identification.
Capillary Electrophoresis-SELEX for High-Affinity aptamers
Capillary Electrophoresis-SELEX (CE-SELEX) is an advanced selection method that combines the principles of SELEX with the high-resolution separation capabilities of capillary electrophoresis. This technique allows for the rapid selection of aptamers with exceptionally high affinity and specificity.
In CE-SELEX, the separation of bound and unbound sequences is achieved based on their differential mobility in an electric field. This method offers several advantages:
- Rapid selection process, often requiring fewer rounds than traditional SELEX
- Ability to select aptamers with very high affinity (Kd values in the picomolar range)
- Minimal consumption of target molecules
- Potential for automation and high-throughput selection
CE-SELEX has been successfully used to generate aptamers against a variety of targets, including proteins, small molecules, and even cell surface markers, making it a valuable tool in the development of precision diagnostic assays.
Microfluidic-based aptamer selection platforms
Microfluidic technologies have revolutionized many aspects of biological research, and aptamer selection is no exception. Microfluidic-based SELEX platforms offer several advantages over traditional methods, including reduced sample consumption, increased automation, and the ability to perform multiple selection steps in a single device.
These platforms typically integrate various functions, such as:
- Sample mixing and incubation
- Target capture and washing
- Aptamer amplification
- Real-time monitoring of the selection process
Microfluidic SELEX can significantly accelerate the aptamer discovery process, potentially reducing the time from target identification to aptamer development from weeks to days. This rapid turnaround is particularly valuable in the context of emerging infectious diseases or personalized medicine applications where quick development of diagnostic tools is crucial.
Aptamer-based biosensors in clinical diagnostics
The integration of labeled aptamers into biosensor platforms has led to the development of highly sensitive and specific diagnostic tools. Aptamer-based biosensors combine the molecular recognition capabilities of aptamers with various transduction mechanisms to convert binding events into measurable signals. These biosensors offer several advantages over traditional diagnostic methods, including rapid detection times, minimal sample preparation, and the potential for multiplexed analysis.
Aptamer-based biosensors can be broadly classified based on their transduction mechanism:
- Electrochemical biosensors
- Optical biosensors
- Mass-sensitive biosensors
- Thermal biosensors
Each type of biosensor has its own strengths and is suited for different diagnostic applications. For example, electrochemical aptasensors are often favored for their simplicity and potential for miniaturization, making them ideal for point-of-care diagnostics. Optical biosensors, on the other hand, can offer exceptional sensitivity and are well-suited for applications requiring multiplexed detection.
The development of aptamer-based biosensors for clinical diagnostics has focused on several key areas:
- Detection of cancer biomarkers
- Monitoring of therapeutic drug levels
- Rapid diagnosis of infectious diseases
- Assessment of cardiovascular health markers
- Detection of allergens and environmental toxins
These biosensors leverage the unique properties of aptamers, such as their ability to undergo conformational changes upon target binding, to create highly sensitive and specific detection systems. By carefully designing the aptamer sequence and labeling strategy, researchers have developed biosensors capable of detecting biomarkers at clinically relevant concentrations in complex biological samples.
Applications of labeled aptamers in molecular imaging
The use of labeled aptamers extends beyond in vitro diagnostics into the realm of molecular imaging. By conjugating aptamers with appropriate imaging agents, researchers have developed powerful tools for visualizing biological processes and disease states in living organisms. This application of aptamer technology has significant implications for both diagnostic and therapeutic monitoring in fields such as oncology, neurology, and cardiovascular medicine.
PET imaging with radiolabeled aptamers
Positron Emission Tomography (PET) is a highly sensitive imaging technique that provides three-dimensional information about metabolic processes in the body. The use of radiolabeled aptamers as PET tracers offers several advantages over traditional small molecule or antibody-based tracers:
- Rapid clearance from the bloodstream, leading to improved signal-to-noise ratios
- High target specificity, reducing background signal
- Ability to engineer aptamers for optimal pharmacokinetics
- Potential for multiplexed imaging using aptamers with different radioisotopes
Researchers have developed radiolabeled aptamers for PET imaging of various targets, including cancer-specific proteins and cell surface markers. These aptamer-based PET tracers have shown promise in preclinical studies for applications such as tumor detection, assessment of treatment response, and monitoring of metastatic spread.
Fluorescence microscopy using aptamer probes
Fluorescently labeled aptamers have found extensive use in both in vitro and in vivo imaging applications. In fluorescence microscopy, aptamer probes offer several advantages over traditional antibody-based staining methods:
- Smaller size, allowing for better tissue penetration
- Reversible binding, enabling dynamic imaging of cellular processes
- Ease of multiplexing with different fluorophores
- Potential for developing activatable probes that fluoresce only upon target binding
These properties have made fluorescent aptamer probes valuable tools for studying cellular processes, tracking protein localization, and investigating drug-target interactions at the single-cell level. In the context of diagnostics, fluorescent aptamer probes have been used for applications such as circulating tumor cell detection and pathogen identification in clinical samples.
MRI contrast enhancement with Aptamer-Conjugated nanoparticles
Magnetic Resonance Imaging (MRI) is a powerful non-invasive imaging technique that provides high-resolution anatomical information. The combination of aptamers with MRI contrast agents, particularly nanoparticle-based contrast agents, has led to the development of targeted imaging probes with enhanced sensitivity and specificity.
Aptamer-conjugated MRI contrast agents typically consist of:
- A magnetic core (e.g., iron oxide nanoparticles)
- A biocompatible coating
- Aptamers for target recognition
- Optional additional functional groups (e.g., therapeutic agents for theranostic applications)
These targeted contrast agents can accumulate at specific sites of interest, such as tumors or sites of inflammation, leading to enhanced contrast and improved diagnostic accuracy. The ability to functionalize nanoparticles with multiple aptamers also opens
the possibility of developing multimodal imaging probes that combine MRI contrast enhancement with other imaging or therapeutic modalities.
Challenges and future directions in aptamer labeling technology
While aptamer labeling technology has made significant strides in enabling precision diagnostics, several challenges remain to be addressed for its widespread adoption in clinical settings. These challenges present opportunities for future research and development in the field.
One of the primary challenges is the stability of aptamers in biological fluids. Although aptamers are generally more stable than antibodies, they can still be susceptible to degradation by nucleases present in blood and other bodily fluids. Researchers are exploring various strategies to enhance aptamer stability, including:
- Chemical modifications of the sugar-phosphate backbone
- Incorporation of non-natural nucleotides
- Encapsulation in protective nanocarriers
- Development of mirror-image L-DNA aptamers resistant to natural nucleases
Another area of focus is improving the pharmacokinetics of labeled aptamers for in vivo applications. The relatively small size of aptamers can lead to rapid renal clearance, which may limit their effectiveness in certain diagnostic or therapeutic applications. Strategies being explored to address this issue include:
- Conjugation with high molecular weight polymers like polyethylene glycol (PEG)
- Incorporation into nanoparticle systems
- Development of aptamer-based scaffolds that increase circulatory half-life
The specificity of aptamers in complex biological environments also remains a challenge. While aptamers can be highly specific under controlled conditions, non-specific binding in the presence of abundant proteins and other biomolecules can reduce their effectiveness. Researchers are working on developing more robust selection methods and optimization techniques to enhance aptamer specificity in real-world diagnostic applications.